Titanium alloys were originally developed in the early 1950s for aerospace
applications, in which their high strength-to-density ratios were
especially attractive. Although titanium alloys are still vital to
the aerospace industry for these properties, recognition of the
excellent resistance of titanium to many highly corrosive environments,
particularly oxidizing and chloride-containing process streams, has
led to widespread non-aerospace (industrial) applications.
Because of decreasing cost and the increasing availability of titanium alloy
products, many titanium alloys have become standard engineering materials
for a host of common industrial applications. In fact, a growing trend
involves the use of high-strength aerospace-founded titanium alloys for
industrial service in which the combination of strength to density and
corrosion resistance properties is critical and desirable.
The excellent corrosion resistance of titanium alloys results from the
formation of very stable, continuous, highly adherent, and protective
oxide films on metal surfaces. Because titanium metal is highly reactive
and has an extremely high affinity for oxygen, these beneficial surface
oxide films form spontaneously and instantly when fresh metal surfaces
are exposed to air and/or moisture. In fact, a damaged oxide film can
generally reheal itself instantaneously if at least traces of oxygen or
water are present in the environment. However, anhydrous conditions in
the absence of a source of oxygen may result in titanium corrosion,
because the protective film may not be regenerated if damaged.
The nature, composition, and thickness of the protective surface oxides
that form on titanium alloys depend on environmental conditions. In most
aqueous environments, the oxide is typically TiO2, but may consist of
mixtures of other titanium oxides, including TiO2,
Ti2O3, and TiO.
High-temperature oxidation tends to promote the formation of the
chemically resistant, highly crystalline form of TiO, known as rutile,
whereas lower temperatures often generate the more amorphous form of TiO,
anatase, or a mixture of rutile and anatase.
Although these naturally formed films are typically less than 10 nm thick
and are invisible to the eye, the TiO; oxide is highly chemically resistant
and is attacked by very few substances, including hot, concentrated HCl,
H2SO4, NaOH, and (most notably) HF. This thin surface oxide
is also a highly effective barrier to hydrogen.
The methods of expanding the corrosion resistance of titanium into
reducing environments include:
- Increasing the surface oxide film thickness by anodizing or thermal oxidation
- Anodically polarizing the alloy (anodic protection) by impressed
anodic current or galvanic coupling with a more noble metal in order to
maintain the surface oxide film
- Applying precious metal (or certain metal oxides) surface coatings
- Alloying titanium with certain elements
- Adding oxidizing species (inhibitors) to the reducing environment to
permit oxide film stabilization
Titanium alloys, like other metals, are subject to corrosion in certain
environments. The primary forms of corrosion that have been observed on
these alloys include general corrosion, crevice corrosion, anodic pitting,
hydrogen damage, and SCC.
In any contemplated application of titanium, its susceptibility to
degradation by any of these forms of corrosion should be considered.
In order to understand the advantages and limitations of titanium alloys,
each of these forms of corrosion will be explained. Although they are not
common limitations to titanium alloy performance, galvanic corrosion,
corrosion fatigue, and erosion-corrosion are included in the interest of
completeness.
General corrosion is characterized by a relatively uniform attack over
the exposed surface of the metal. At times, general corrosion in aqueous
media may take the form of mottled, severely roughened metal surfaces that
resemble localized attack. This often results from variations in the
corrosion rates of localized surface patches due to localized masking
of metal surfaces by process scales, corrosion products, or gas bubbles;
such localized masking can prevent true uniform surface attack.
Titanium alloys may be subject to localized attack in tight crevices
exposed to hot (>70 oC) chloride, bromide, iodide, fluoride,
or sulfate-containing solutions. Crevices can stem from adhering
process stream deposits or scales, metal-to-metal joints (for example,
poor weld joint design or tube-to-tubesheet joints), and gasket-to-metal
flange and other seal joints.
Pitting is defined as localized corrosion attack occurring on openly
exposed metal surfaces in the absence of any apparent crevices.
This pitting occurs when the potential of the metal exceeds the
anodic breakdown potential of the metal oxide film in a given environment.
When the anodic breakdown potential of the metal is equal to or less than
the corrosion potential under a given set of conditions, spontaneous
pitting can be expected.
Titanium alloys are widely used in hydrogen containing environments and
under conditions in which galvanic couples or cathodic charging causes
hydrogen to be evolved on metal surfaces. Although excellent performance
is revealed for these alloys in most cases, hydrogen embrittlement has
been observed.
The surface oxide film of titanium is a highly effective barrier to
hydrogen penetration. Traces of moisture or oxygen in hydrogen-containing
environments very effectively maintain this protective film, thus
avoiding or limiting hydrogen uptake. On the other hand, anhydrous
hydrogen gas atmospheres may lead to absorption, particularly as
temperatures and pressures increase.
Stress-corrosion cracking (SCC) is a fracture, or cracking, phenomenon
caused by the combined action of tensile stress, a susceptible alloy,
and a corrosive environment. The metal normally shows no evidence of
general corrosion attack, although slight localized attack in the form
of pitting may be visible. Usually, only specific combinations of
metallurgical and environmental conditions cause SCC. This is important
because it is often possible to eliminate or reduce SCC sensitivity by
modifying either the metallurgical characteristics of the metal or the
makeup of the environment.
Another important characteristic of SCC is the requirement that tensile
stress is present. These stresses may be provided by cold work, residual
stresses from fabrication, or externally applied loads.
The key to understanding SCC of titanium alloys is the observation that
no apparent corrosion, either uniform or localized, usually precedes
the cracking process. As a result, it can sometimes be difficult to
initiate cracking in laboratory tests by using conventional test techniques.
It is also important to distinguish between the two classes of titanium
alloys. The first class, which includes ASTM grades 1, 2, 7, 11 and 12,
is immune to SCC except in a few specific environments. These specific
environments include anhydrous methanol/halide solutions, nitrogen
tetroxide (N2O4), and liquid or solid cadmium. The second class of
titanium alloys, including the aerospace titanium alloys, has been
found to be susceptible to several additional environments, most
notably aqueous chloride solutions.
The coupling of titanium with dissimilar metals usually does not
accelerate the corrosion of titanium. The exception is in strongly
reducing environments in which titanium is severely corroding and not
readily passivated. In this uncommon situation, accelerated corrosion
may occur when titanium is coupled to more noble metals. In its normal
passive condition, materials that exhibit more noble corrosion potentials
beneficially influence titanium.
The general corrosion resistance of titanium can be improved or expanded
by one or a combination of the following strategies:
- Alloying
- Inhibitor additions to the environment
- Precious metal surface treatments
- Thermal oxidation
- Anodic protection.
Alloying. Perhaps the most effective and preferred means of extending
resistance to general corrosion in reducing environments has been by
alloying titanium with certain elements. Beneficial alloying elements
include precious metals (>0.05 wt% Pd), nickel ( >= 0.5 wt%), and/or
molybdenum (>= 4 wt%). These additions facilitate cathodic depolarization
by providing sites of low hydrogen overvoltage, which shifts alloy
potential in the noble direction where oxide film passivation is possible.
Relatively small concentrations of certain precious metals (of the order
of 0.1 wt%) are sufficient to expand significantly the corrosion resistance
of titanium in reducing acid media.
These beneficial alloying additions have been incorporated into several
commercially available titanium alloys, including the titanium-palladium
alloys (grades 7 and 11), Ti-0.3Mo-0.8Ni (grade 12), Ti-3Al-8V-6Cr-4Zr-4Mo,
Ti-15Mo-5Zr, and Ti-6Al-2Sn-4Zr-6Mo. These alloys all offer expanded
application into hotter and/or stronger HCl, H2SO4,
H3PO4, and other reducing acids as compared
to unalloyed titanium. The high-molybdenum
alloys offer a unique combination of high strength, low density, and
superior corrosion resistance.
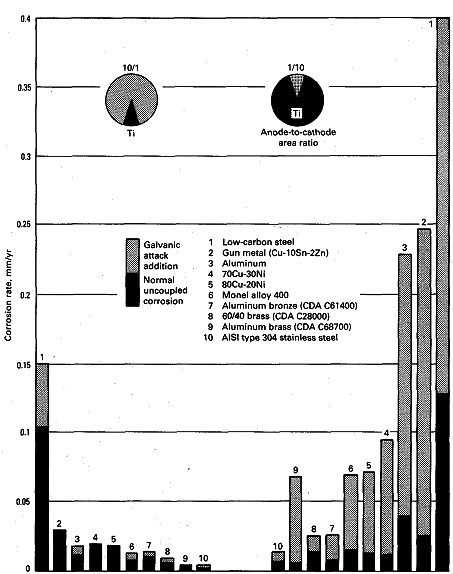 |
Fig 1. Corrosion of dissimilar metals coupled to
titanium in flowing ambient-temperature seawater |
List of Articles - Knowledge Base