Manganese
All commercial steels
contain 0,3-0,8% manganese, to reduce oxides and to counteract the harmful
influence of iron sulfide. Any manganese in excess of
these requirements partially dissolves in the iron and partly form Mn3C which
occurs with the Fe3C. There is a tendency nowadays to increase the manganese
content and reduce the carbon content in order to get
a steel with an equal tensile strength but improved ductility
If the manganese is
increased above 1,8% the steel tends to become airhardened, with resultant
impairing of the ductility. Up to this quantity, manganese has a
beneficial effect on the mechanical properties of oil hardened
and tempered 0,4% carbon steel. The manganese content is also increased in
certain alloy steels, with a reduction or elimination of expensive nickel, in order
to reduce costs. Steels with 0,3-0,4% carbon, 1,3-1,6% manganese and
0,3% molybdenum have replaced 3% nickel steel for some purposes.
Non-shrinking tool steel contains up to 2%
manganese, with 0,8-0,9% carbon. Steels with 5 to 12% manganese
are martensitic after slow cooling and have little commercial importance.
Hadfield`s manganese steel contains 12 to 14%
of manganese and 1,0% of carbon. It is characterized by a great
resistance to wear and is therefore used for railway points, rock drills
and stone crushers. Austenite is completely retained by quenching
the steel from 1000°C, in which soft condition it is used,
but abrasion raises the hardness of the surface layer from 200
to 600 VPN (with no magnetic change), while the
underlying material remains rough. Annealing embrittles the steel by the formation of
carbides at the grain boundaries. Nickel is added to electrodes for welding manganese
steel and 2% Mo sometimes added, with a prior carbide
dispersion treatment at 600°C, to minimize initial distortion and spreading.
Nickel
Nickel and manganese are very similar in
behavior and both lower the eutectoid temperature. This change point
on heating is lowered progressively with increase of nickel
(approximately 10°C for 1% of nickel), but the lowering of the
change on cooling is greater and irregular. The temperature of this
change (Ar1) is plotted for different nickel contents for 0,2%
carbon steels in Fig. 1, and it will be seen that the curve takes a
sudden plunge round about 8% nickel. A steel with 12% nickel begins
to transform below 300°C on cooling, but on reheating the reverse
change does not occur until about 650°C. Such steels are said to
exhibit pronounced lag or hysteresis and are called irreversible steels. This characteristic is made use of in maraging
steels and 9% Ni cryogenic steel.
The addition of nickel acts similarly to increasing the rate of cooling of a
carbon steel. Thus with a constant rate of cooling the 5-8%
nickel steels become troostitic; at 8-10% nickel, where the
sudden drop appears, the structure is martensitic, while above 24% nickel the
critical point is depressed below room temperature and austenite remains. The lines of
demarcation are not so sharp as indicated by Fig. 1,
but a gradual transition occurs from one constituent to another.
Figure 1. Effect of nickel on change points and mechanical
properties of 0,2% carbon steels cooled at a constant rate
The mechanical properties change
accordingly as shown in the lower part of Fig. 1. Steels with 0,5%
nickel are similar to carbon steel, but are stronger, on account of
the finer pearlite formed and the presence of nickel in solution in
the ferrite. When 10% nickel is exceeded the steels have a high
tensile strength, great hardness, but are brittle, as shown by the
Izod and elongation curves. When the nickel is sufficient to produce
austenite the steels become non-magnetic, ductile, tough and
workable, with a drop in strength and elastic limit.
Carbon intensives the action of nickel and the change points shown in Fig.
1 will vary according to the carbon content. The influence of carbon
and nickel on the structure are shown in the small inset (Guillet)
diagram in Fig. 1, for one rate of cooling. Steels containing 2 to
5% nickel and about 0,1% carbon are used for case hardening; those
containing 0,25 to 0,40% carbon are used for crankshafts, axles and
connecting rods.
The superior properties of low nickel steels
are best brought out by quenching and tempering (550-650°C). Since
the Ac3 point is lowered, a lower hardening temperature than for
carbon steels is permissible and also a wider range of hardening
temperatures above Ac3 without excessive grain growth, which is
hindered by the slow rate of diffusion of the nickel. Martensitic
nickel steels are not utilized and the austenitic alloys cannot
compete with similar manganese steels owing to the higher cost.
Maraging steels have fulfilled a high tensile requirement in aero
and space fields. High nickel alloys are used for special purposes,
owing to the marked influence of nickel on the coefficient of
expansion of the metal. With 36% nickel, 0,2% carbon, 0,5%
manganese, the coefficient is practically zero between 0° and 100°C.
This alloy ages with time, but this can be minimized by heating at
100°C for several days. The alloy is called Inver and it is used
extensively in clocks, tapes and wire measures, differential
expansion regulators, and in aluminium pistons with a split skirt in
order to give an expansion approximating to that of cast iron.
A carbon-free alloy containing 78,5% nickel and
21,5% iron has a high permeability in small magnetic fields.
Chromium
Chromium can dissolve in either
alpha- or gama-iron, but, in the presence of carbon, the carbides
formed are cementite (FeCr)3C in which chromium may rise to more
than 15%; chromium carbides (CrFe)3C2 (CrFe)7C3 (CrFe)4C, in which
chromium may be replaced by a few per cent, by a maximum
of 55% and by 25% respectively. Stainless steels contain Cr4C. The pearlitic chromium
steels with, say, 2% chromium are extremely sensitive to rate
of cooling and temperature of heating before quenching; for example:
Temp. of Initial Heating, °C |
Critical Hardening Rate (Mins to cool from
836° to 546°C) |
836 |
3,5 |
1010 |
6,5 |
1200 |
13 |
The reason is that the chromium carbides are
not readily dissolved in the austenite, but the amount increases
with increase of temperature. The effect of the dissolved chromium
is to raise the critical points on heating (Ac) and also on cooling
(Ar) when the rate is slow. Faster rates of cooling quickly depress
the Ar points with consequent hardening of the steel. Chromium
imparts a characteristic form of the upper portion of the isothermal
transformation curve.
The percentage of carbon in the pearlite
is lowered. Hence the proportion of free cementite (hardest
constituent) is increased in high carbon steel and, when the steel
is properly heat-treated, it occurs in the spheroidised form which
is more suitable when the steel is used for ball bearings. The
pearlite is rendered fine.
When the chromium exceeds 1,1% in
low-carbon steels an inert passive film is formed on the surface
which resists attack by oxidizing reagents. Still higher chromium
contents are found in heat-resisting steel.
Chromium steels are
easier to machine than nickel steels of similar tensile strength.
The steels of higher chromium contents are susceptible to temper
brittleness if slowly cooled from the tempering temperature through
the range 550/450°C. These steels are also liable to form surface
markings, generally referred to as "chrome lines".
The chrome steels are used wherever extreme
hardness is required, such as in dies, ball bearings, plates for
safes, rolls, files and tools. High chromium content is also found
in certain permanent magnets.
Nickel and chromium
Nickel steels are noted for their strength,
ductility and toughness, while chromium steels are characterized by
their hardness and resistance to wear. The combination of nickel and
chromium produces steels having all these properties, some
intensified, without the disadvantages associated with the simple
alloys. The depth of hardening is increased, and with 4,5% nickel,
1,25% chromium and 0,35% carbon the steel can be hardened simply by
cooling in air.
Low nickel-chromium steels with small carbon
content are used for casehardening, while for most constructional
purposes the carbon content is 0,25-0,35%, and the steels are
heat-treated to give the desired properties. Considerable amounts of
nickel and chromium are used in steel for resisting corrosion and
oxidation at elevated temperatures.
Embattlement. The effects of
tempering a nickel-chromium steel are shown in Fig. 2, from which it
will be noticed that the Izod impact curve No. 1 reaches a dangerous
minimum in the range 250-450°C in common with many other steels.
This is known as 350°C embattlement. Phosphorus and nitrogen have a
significant effect while other impurities (As, Sb, Sn) and manganese
in larger quantity may also contribute to the embattlement.
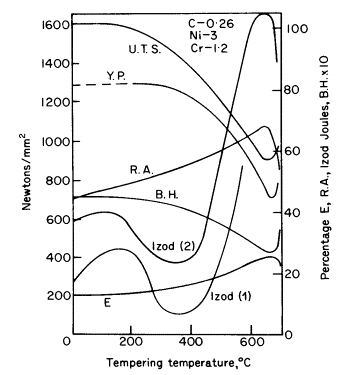
Figure 2. Effect of tempering on
the mechanical properties of nickel-chromium steel, C 0,26, Ni 3, Cr
1,2, 29 mm diam, bars hardened in oil from 830°C. Izod (2) for steel
with 0,25% molybdenum added
Temper brittleness is
usually used to describe the notch impact intergranular brittleness
(Grain boundaries are revealed in temper brittle samples by etching
in 1 gm cetyl trimethyl ammonium bromide; 20 gm picric acid; 100 cc
distilled water, 100 cc ether. Shake mixture, allow to stand for 24
hrs; use portion of top layer and return to tube afterwards) induced
in some steels by slow cooling after tempering above about
600°C and also from prolonged soaking of tough material between
about 400° and 550°C.
Temper brittleness seems to be due to grain
boundary enrichment with alloying elements-Mn, Cr, Mo-during
austenitising which leads to enhanced segregation of embattling
elements P, Sn, Sb, As-by chemical interaction on slow cooling from
600°C. The return to the tough condition, obtained by rehearing
embattled steel to temperatures above 600°C and rapidly
cooling, is due to the redistribution and retention in solution
of the embattling segregation. Antimony (0-001 %), phosphorus (0-008
%), arsenic, tin, manganese increase, while molybdenum decreases
the susceptibility of a steel to embattlement. 0-25 %
molybdenum reduces the brittleness as shown by Izod curve No. 2.
Table 1 illustrates the effect rate of cooling after tempering and
the influence of an addition of 0-45 % molybdenum:
Table 1. Steel 0,3% C, 3,5 % Ni,
0,7%, Cr, tempered at 630°C
Steel |
Cooling Rate |
TS MPa |
Elongation |
RA |
Izod ft lbf |
Izod J |
Ni-Cr |
Oil |
896 |
18 |
60 |
64 |
87 |
Ni-Cr |
Furnance |
880 |
18 |
60 |
19 |
25 |
Ni-Cr-Mo |
Furnance |
896 |
18 |
61 |
59 |
80 |
Molybdenum
Molybdenum dissolves in both alpha- and gama-iron
and in the presence of carbon forms complex carbides (FeMo)6C,
Fe21Mo2C6, Mo2C.
Molybdenum is similar to chromium in its effect
on the shape of the TTT-curve but up to 0,5% appears to be more
effective in retarding pearlite and increasing bainite formation.
Additions of 0,5% molybdenum have been made to plain carbon steels
to give increased strength at boiler temperatures of 400°C, but the
element is mainly used in combination with other alloying elements.
Ni-Cr-Mo steels are widely used for ordnance, turbine rotors and
other large articles, since molybdenum tends to minimize temper
brittleness and reduces mass effect. Molybdenum is also a
constituent in some high-speed steels, magnet alloys, heat-resisting
and corrosion-resisting steels.
Vanadium
Vanadium acts as a scavenger for oxides,
forms a carbide V,C, and has a beneficial effect on the mechanical
properties of heat-treated steels, especially in the presence of
other elements. It slows up tempering in the range of 500-600°C and
can induce secondary hardening. Chromium-vanadium (0,15%) steels are
used for locomotive forging, automobile axles, coil springs, torsion
bars and creep resistance.
Tungsten
Tungsten dissolves in gama-iron and in alpha-iron.
With carbon it forms WC and W2C, but in the presence of iron it
forms Fe3W3C or Fe4W2C. A compound with iron-Fe3W2-provides an
age-hardening system. Tungsten raises the critical points in steel
and the carbides dissolve slowly over a range of temperature. When
completely dissolved, the tungsten renders transformation sluggish,
especially to tempering, and use is made of this in most hot-working
tool ("high speed") and die steels. Tungsten refines the grain size
and produces less tendency to decarburisation during working.
Tungsten is also used in magnet, corrosion- and heat-resisting
steels.
Silicon
Silicon dissolves in the ferrite, of which it
is a fairly effective hardener, and raises the Ac change points and
the Ar points when slowly cooled and also reduces the gama-alpha volume
change.
Only three types of silicon steel are in common use-one
in conjunction with manganese for springs; the second for electrical
purposes, used in sheet form for the construction of transformer
cores, and poles of dynamos and motors, that demand high magnetic
permeability and electrical resistance; and the third is used for
automobile valves.
|
C |
Si |
Mn |
1. Silico-manganese |
0,5 |
1,5 |
0,8 |
2. Silicon steel |
0,07 |
4,3 |
0,09 |
3. Silichrome |
0,4 |
3,5 |
8 |
It contributes oxidation resistance in heat-resisting steels and
is a general purpose deoxidizes.
Other elements
Copper dissolves in the ferrite to a limited extent; not
more than 3,5% is soluble in steels at normalizing temperatures,
while at room temperature the ferrite is saturated at 0,35%. It
lowers the critical points, but insufficiently to produce martensite
by air cooling. The resistance to atmospheric corrosion is improved
and copper steels can be temper hardened.
Cobalt has a high solubility in alpha- and gama-iron but a weak
carbide-forming tendency. It decreases hardenability but sustains
hardness during tempering. It is used in "Stellite" type alloys, gas
turbine steel, magnets and as a bond in hard metal.
Boron. In recent years, especially in USA, 0,003-0,005%
boron has been added to previously fully killed, fine-grain steel to
increase the hardenability of the steel. The yield ratio and impact
are definitely improved, provided advantage is taken of the
increased hardenability obtained and the steel is fully hardened
before tempering. In conjunction with molybdenum boron forms a
useful group of high tensile bainitic steels. Boron is used in some
hard facing alloys and for nuclear control rods.
List of Articles - Knowledge Base