Hardening of steel is obtained by a suitable quench from
within or above the critical range. The temperatures are the
same as those given for full annealing. The soaking time in air furnaces
should be 1,2 min for each mm of cross-section or 0,6 min in
salt or lead baths. Uneven heating, overheating and excessive scaling
should be avoided.
The quenching is necessary to suppress the normal breakdown of
austenite into ferrite and cementite, and to cause a partial decomposition
at such a low temperature to produce martensite. To obtain this, steel
requires a critical cooling velocity, which is greatly reduced by
the presence of alloying elements, which therefore cause hardening with
mild quenching (e.g. oil and hardening steels).
Steels with less than 0,3 % carbon cannot be hardened effectively,
while the maximum effect is obtained at about 0,7 % due to an increased
tendency to retain austenite in high carbon steels Fig. 1.
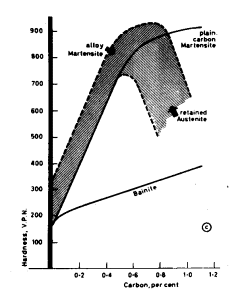
Figure 1. Variation of hardness of martensite and bainite
with carbon content
Water is one of the most efficient quenching media where
maximum hardness is required, but it is liable to cause distortion and
cracking of the article. Where hardness can be sacrificed, whale, cotton
seed and mineral oils are used. These tend to oxidise and form sludge with
consequent lowering of efficiency.
The quenching velocity of oil is much less than water.
Ferrite and troostite are formed even in small sections. Intermediate
rates between water and oil can be obtained with water containing 10-30 %
Ucon, a substance with an inverse solubility which therefore deposits on
the object to slow rate of cooling. To minimise distortion, long
cylindrical objects should be quenched vertically, flat sections edgeways
and thick sections should enter the bath first. To prevent steam bubbles
forming soft spots, a water quenching bath should be agitated.
Fully hardened and tempered steels develop the best
combination of strength and notch-ductility.
Tempering and toughening
The martensite of quenched tool steel is exceedingly brittle
and highly stressed. Consequently cracking and distortion of the object
are liable to occur after quenching. Retained austenite is unstable and as
it changes dimensions may alter, e.g. dies may alter 0,012 mm.
It is necessary, therefore, to warm the steel below the
critical range in order to relieve stresses and to allow the arrested
reaction of cementite precipitation to take place. This is known as
tempering.
-
150-250°C. The object is heated in an oil bath,
immediately after quenching, to prevent related cracking, to relieve
internal stress and to decompose austenite without much
softening.
-
200-450°C. Used to toughen the steel at the expense of
hardness. Brinell hardness is 350-450.
-
450-700°C. The precipitated cementite coalesces into
larger masses and the steel becomes softer. The structure is known as
sorbite, which at the higher temperatures becomes coarsely spheroidised.
It etches more slowly than troostite and has a Brinell hardness of
220-350. Sorbite is commonly found in heat-treated constructional
steels, such as axles, shafts and crankshafts subjected to dynamic
stresses. A treatment of quenching and tempering in this temperature
range is frequently referred to as toughening, and it produces an
increase in the ratio of the elastic limit to the ultimate tensile
strength.
The reactions in tempering occur slowly. Reaction time as
well as temperature of heating is important. Tempering is carried out to
an increasing extent under pyrometric control in oil, salt (e.g. equal
parts sodium and potassium nitrates for 200-600°C) or lead baths and also
in furnaces in which the air is circulated by fans. After the tempering,
the objects may be cooled either rapidly or slowly, except for steels
susceptible to temper brittleness.
Temper colours formed on a cleaned surface are still used
occasionally as a guide to temperature. They exist due to the interference
effects of thin films of oxide formed during tempering, and they act
similarly to oil films on water. Alloys such as stainless steel form
thinner films than do carbon steels for a given temperature and hence
produce a colour lower in the series. For example, pale straw corresponds
to 300°C, instead of 230°C (Table 1).
Table 1.
Temper Colour |
Temperature °C |
Objects |
Pale straw |
230 |
Planing and slotting tools |
Dark straw |
240 |
Milling cutters, drills |
Brown |
250 |
Taps, shear blades for metals |
Brownish-purple |
260 |
Punches, cups, snaps, twist drills, reamers |
Purple |
270 |
Press tools, axes |
Dark purple |
280 |
Cold chisels, setts for steel |
Blue |
300 |
Saws for wood, springs |
Blue |
450-650 |
Toughening for constructional steels |
For turning, planing, shaping tools and
chisels, only the cutting parts need hardening. This is frequently carried
out in engineering works by heating the tool to 730°C, followed by
quenching the cutting end vertically. When cutting end gets cold, it is
cleaned with the stone and the heat from the shank of the tool is allowed
to temper the cutting edge to the correct colour. Then the whole tool is
quenched. Oxidation can be reduced by coating the tool with charcoal and
oil.
Changes during tempering
The principles underlying the tempering of quenched steels
have a close similarity to those of precipitation hardening. The
overlapping changes, which occur when high carbon martensite is tempered,
are shown in Fig. 2 and as follows:
-
Stage 1. 50-200°C. Martensite breaks
down to a transition precipitate known as c-carbide (Fe2,4C) across
twins and a low carbon martensite which results in slight dispersion
hardening, decrease in volume and electrical resistance.
-
Stage 2. 205-305°C. Decomposition of
retained austenite to bainite and decrease in hardness.
-
Stage 3. 250-500°C. Conversion of the
aggregate of low carbon martensite and c-carbide into ferrite and
cementite precipitated along twins, which gradually coarsens to give
visible particles and rapid softening, Fig. 3.
-
Stage 4. Carbide changes in alloy steel
at 400-700°C. In steels containing one alloying addition, cementite
forms first and the alloy diffuses to it. When sufficiently enriched the
Fe3C transforms to an alloy carbide. After further enrichment this
carbide may be superseded by another and this formation of transition
carbides may be repeated several times before the equilibrium carbide
forms. In chromium steel, changes are: Fe3C®Cr7C3®Cr23C6. In steels
containing several carbide-forming elements the reactions are often more
complex, and the carbides which decompose are not necessarily followed
by carbides based on the same alloy elements. The transformation can
also occur in situ by gradual exchange of atoms without any appreciable
hardening; or by resolution of existing iron carbides and fresh
nucleation of coherent carbide with considerable hardening that
counteracts the normal softening that occurs during tempering. In some
alloy steels, therefore, the hardness is maintained constant up to about
500°C or in some cases it rises to a peak followed by a gradual drop due
to breakdown of coherence and coalescence of the carbide particles. This
age-hardening process is known as secondary hardening and it enhances
high temperature creep properties of steel (e.g. steel E in Fig. 2).
Chromium, for an example, seems to stabilise the size of the cementite
particles over a range 200-500°C. Vanadium and molybdenum form a fine
dispersion of coherent precipitates
(V4C3Mo2C) in a ferrite matrix with
considerable hardening. When over-ageing starts the
V4C3 grows in the grain boundaries and also forms
a Widmanstätten pattern of plates within the grain.
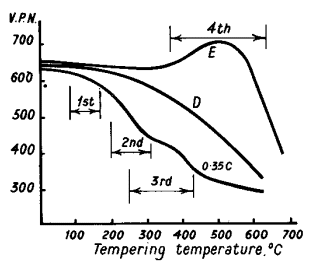
Figure 2.Tempering curves for 0,35 % C steel and die
steel
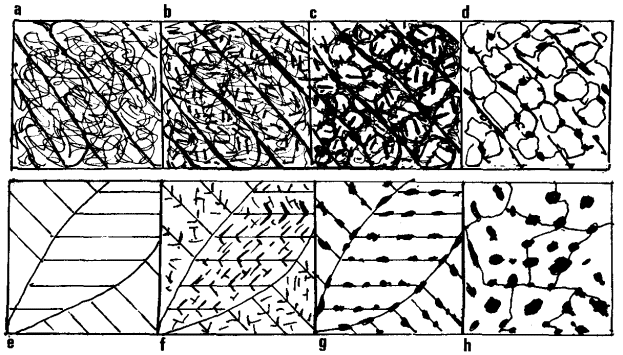
a) As quenched. Laths with high density of dislocation |
b) Tempered 300°C. Widmanstätten precipitation of carbides
within laths |
c) Tempered 500°C. Recovery of dislocation structure into cells
with laths |
d) Tempered 600°C. Recrystallisation cemen-tite re-nucleated
equioxed ferrite boundaries |
e) High C twinned martensite |
f) Tempered 100°C. Fine e-carbides across twins |
g) Tempered 200°C. Coherent cementite along twins. c-carbides
dissolve |
h) Tempered 400°C. Breakdown of twinned structure. Carbides grow
and spheroidise |
Figure 3. Low carbon lath martensites have a high Ms temperature and
some tempering often occurs on cooling, i.e. autotempering.
Tempering at 300°C causes precipitation of carbides within
the laths in Widmanstätten form (Fig. 3). Tempering at 500°C promotes the
recovery of the dislocation tangle into cells within the laths with
carbides precipitated along boundaries. Tempering at 600°C gives rise to
recrystallisation into equioxed ferrite with carbides re-nucleated at the
boundaries.
Quench cracks
The volume changes, which occur when austenite is cooled,
are: a) expansion when gamma iron transforms to ferrite; b) contraction
when cementite is precipitated; c) normal thermal contraction.
When steel is quenched these volume changes occur very
rapidly and unevenly throughout the specimen. The outside cools most
quickly, and is mainly martensitic, in which contraction (b) has not
occurred. The centre may be troostitic and contraction (b) started.
Stresses are set up which may cause the metal either to
distort or to crack if the ductility is insufficient for plastic flow to
occur. Such cracks may occur some time after the quenching or in the early
stages of tempering.
Quench cracks are liable to occur:
a) due to presence of
non-metallic inclusions, cementite masses, etc.;
b) when austenite is
coarse grained due to high quenching temperature;
c) owing to uneven
quenching;
d) in pieces of irregular section and when sharp re-entrant
angles are present in the design.
The relation of design to heat-treatment is very
important. Articles of irregular section need special care. When steel has
been chosen which needs a water-quench, then the designer must use
generous fillets in the corners and a uniform section should be aimed at.
This can sometimes be obtained by boring out metal from bulky parts
without materially affecting the design; examples are given in Fig.
4.
A hole drilled from the side to meet a central hole may
cause cracking and it should be drilled right through and temporarily
stopped up with asbestos wool during heat-treatment. A crack would also
form at the junction of the solid gear with the shaft. There is a serious
danger of cracks at the roots of the teeth, owing to the great change in
size of section. This design could be improved by machining the metal away
under the rim to make a cross-section of uniform mass.
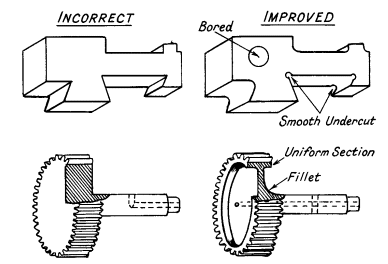
Figure 4. The relation of design to heat-treatment
Fundamentals of heat-treatment
Heat-treatment of steel involves the change of austenite, a
face-centred cubic iron lattice containing carbon atoms in the
interstices, into a body-centred cubic ferrite with a low solubility for
carbon.
The carbon atoms segregate into areas to form cementite.
This involves mobility or diffusion of the carbon atoms and both time and
temperature are important. Atomic movements are rapid at high temperatures
but increasingly sluggish as the temperature decreases.
As the rate of cooling of an austenitised steel increases
the time allowed for the changes is shortened and the reactions are
incomplete at 600-700°C. Residual austenite, therefore, transforms at
lower temperatures, with shorter movements of atoms and finer structures.
At temperatures below about 250°C diffusion is so slow that another
transition structure is formed.
The effect of rapid cooling on the critical points is
complex (Fig. 5). Increase in the rate of cooling has the following
effects:
1. Arrest temperatures are depressed.
2. Ar3 merges
with Ar1 producing a single depressed point known as Ar". Fine
laminated troostite is formed.
3. Accelerated cooling causes another
arrest to appear at 350-150°C, known as Ar". Troostite and martensite
are formed.
4. Rapid quenching causes Ar" to merge into Ar".
Martensite is formed.
5. The arrest due to the formation of bainite
at 500-250°C does not usually appear with carbon steel, but is present
with many alloy steels.
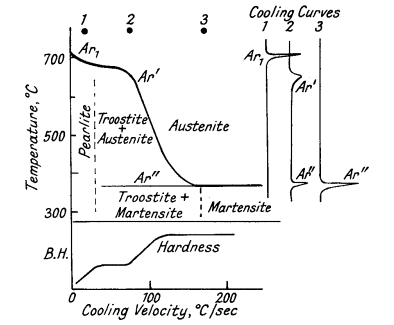
Figure 5. Effect of cooling rate on the transformation
of austenite
List of Articles - Knowledge Base