It is a long-standing tradition to discuss the various alloying elements
in terms of the properties they confer on steel. For example, the rule was
that Chromium (Cr) makes steel hard whereas Nickel (Ni) and Manganese (Mn)
make it tough. In saying this, one had certain types of steel in mind and
transferred the properties of particular steel to the alloying element
that was thought to have the greatest influence on the steel under
consideration. This method of reasoning can give false impressions and the
following examples will illustrate this point.
When we say that Cr makes steel hard and wear-resisting we probably
associate this with the 2% C, 12% Cr tool steel grade, which
on hardening does in fact become very hard and hard-wearing. But if, on the
other hand, we choose a steel containing 0,10% C and 12% Cr,
the hardness obtained on hardening is very modest.
It is quite true that Mn increases steel toughness if we have in
mind the 13% manganese steel, so-called Hadfield steel. In concentrations
between l% and 5%, however, Mn can produce a variable effect on the
properties of the steel it is alloyed with. The toughness may either
increase or decrease.
A property of great importance is the ability of alloying elements to
promote the formation of a certain phase or to stabilize it. These elements
are grouped as austenite-forming, ferrite-forming, carbide-forming and
nitride-forming elements.
Austenite-forming elements
The elements C, Ni and Mn are the most important ones
in this group. Sufficiently large amounts of Ni or Mn render
a steel austenitic even at room temperature. An example of this is the
so-called Hadfield steel which contains 13% Mn, 1,2% Cr and
l% C. In this steel both the Mn and C take part in
stabilizing the austenite. Another example is austenitic stainless
steel containing 18% Cr and 8% Ni.
The equilibrium diagram for iron-nickel, Figure 1, shows how the range of
stability of austenite increases with increasing Ni-content.
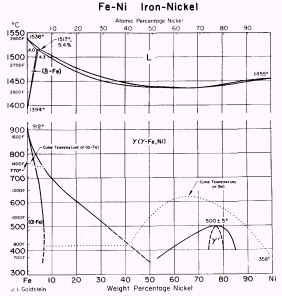
Figure 1. Fe-Ni equilibrium diagram
An alloy containing 10% Ni becomes wholly austenitic if heated to 700°C.
On cooling, transformation from g to a takes place in the temperature
range 700-300°C.
Ferrite-forming elements
The most important elements in this group are Cr, Si,
Mo, W and Al. The range of stability of ferrite in
iron-chromium alloys is shown in Figure 2. Fe-Cr alloys in the solid state
containing more than 13% Cr are ferritic at all temperatures up to
incipient melting. Another instance of ferritic steel is one that is used
as transformer sheet material. This is a low-carbon steel containing about
3% Si.
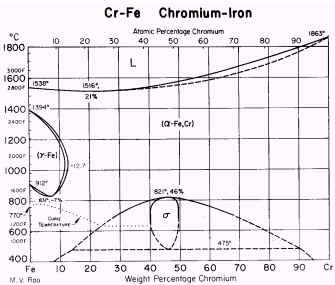
Figure 2. Cr-Fe equilibrium diagram
Multi-alloyed steels
The great majority of steels contain at least three components. The
constitution of such steels can be deduced from ternary phase diagrams
(3 components). The interpretation of these diagrams is relatively
difficult and they are of limited value to people dealing with practical
heat treatment since they represent equilibrium conditions only.
Furthermore, since most alloys contain more than three components it is
necessary to look for other ways of assessing the effect produced by the
alloying elements on the structural transformations occurring during heat
treatment.
One approach that is quite good is the use of Schaeffler diagrams (see
Figure 3). Here the austenite formers are set out along the ordinate and
the ferrite formers along the abscissa. The original diagram contained
only Ni and Cr but the modified diagram includes other
elements and gives them coefficients that reduce them to the equivalents
of Ni or Cr respectively. The diagram holds good for the
rates of cooling which result from welding.
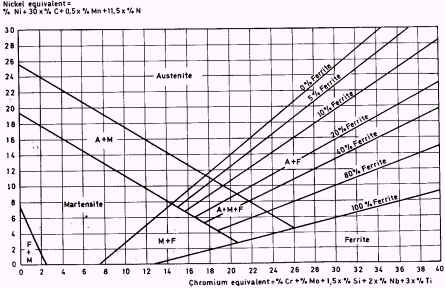
Figure 3. Modified Schaeffler diagram
A 12% Cr steel containing 0,3% C is martensitic, the 0,3%
C gives the steel a nickel equivalent of 9. An 18/8 steel
(18% Cr, 8% Ni) is austenitic if it contains 0-0,5% C
and 2% Mn. The Ni content of such steels is usually kept
between 9% and 10%.
Hadfield steel with 13% Mn (mentioned above) is austenitic due to
its high carbon content. Should this be reduced to about 0,20% the steel
becomes martensitic.
Carbide-forming elements
Several ferrite formers also function as carbide formers. The majority of
carbide formers are also ferrite formers with respect to Fe. The
affinity of the elements in the line below for carbon increases from left
to right.
Cr, W, Mo, V, Ti, Nb, Ta, Zr.
Some carbides may be referred to as special carbides, i.e.
non-iron-containing carbides, such as Cr7C3 W2C, VC, Mo2C. Double or
complex carbides contain both Fe and a carbide-forming element, for
example Fe4W2C.
High-speed and hot-work tool steels normally contain three types of
carbides, which are usually designated M6C, M23C6 and MC. The letter
M represents collectively all the metal atoms. Thus M6C represents Fe4W2C
or Fe4Mo2C; M23C6 represents Cr23C6 and MC represents VC or V4C3.
Carbide stabilizers
The stability of the carbides is dependent on the presence of other
elements in the steel. How stable the carbides are depends on how the
element is partitioned between the cementite and the matrix. The ratio of
the percentage, by weight, of the element contained in each of the two
phases is called the partition coefficient K. The following values are
given for K:
Al
|
Cu
|
P
|
Si
|
Co
|
Ni
|
W
|
Mo
|
Mn
|
Cr
|
Ti
|
Nb
|
Ta
|
0
|
0
|
0
|
0
|
0,2
|
0,3
|
2
|
8
|
11,4
|
28
|
Increasing
|
Note that Mn, which by itself is a very weak carbide former, is a
relatively potent carbide stabilizer. In practice, Cr is the
alloying element most commonly used as a carbide stabilizer.
Malleable cast iron (i.e. white cast iron that is rendered soft by a
graphitizing heat treatment called malleablizing) must not contain any
Cr. Steel containing only Si or Ni is susceptible to
graphitization, but this is most simply prevented by alloying with Cr.
Nitride-forming elements
All carbide formers are also nitride formers. Nitrogen may be introduced
into the surface of the steel by nitriding.
By measuring the hardness of various nitrided alloy steels it is possible
to investigate the tendency of the different alloying elements to form hard
nitrides or to increase the hardness of the steel by a mechanism known as
precipitation hardening.
The results obtained by such investigations are shown in Figure 4, from
which it can be seen that very high hardnesses result from alloying a
steel with Al or Ti in amounts of about 1,5%.
Figure 4.
|
Effect of alloying element additions on hardness after nitriding
Base composition: 0,25% C, 0,30% Si, 0,70% Mn
|
On nitriding the base material in Figure 4, hardness of about 400 HV is
obtained and according to the diagram the hardness is unchanged if the
steel is alloyed with Ni since this element is not a nitride former and
hence does not contribute to any hardness increase.
List of Articles - Knowledge Base